I got it to work and amplify, but the loop gain left a lot to be desired, so I decided to start over on the compensation. I also hooked up an unregulated power supply and a different output stage, partly because I wanted to see how the PSRR was doing, and partly because I wanted to reassemble the Ice Block with its original driver board.
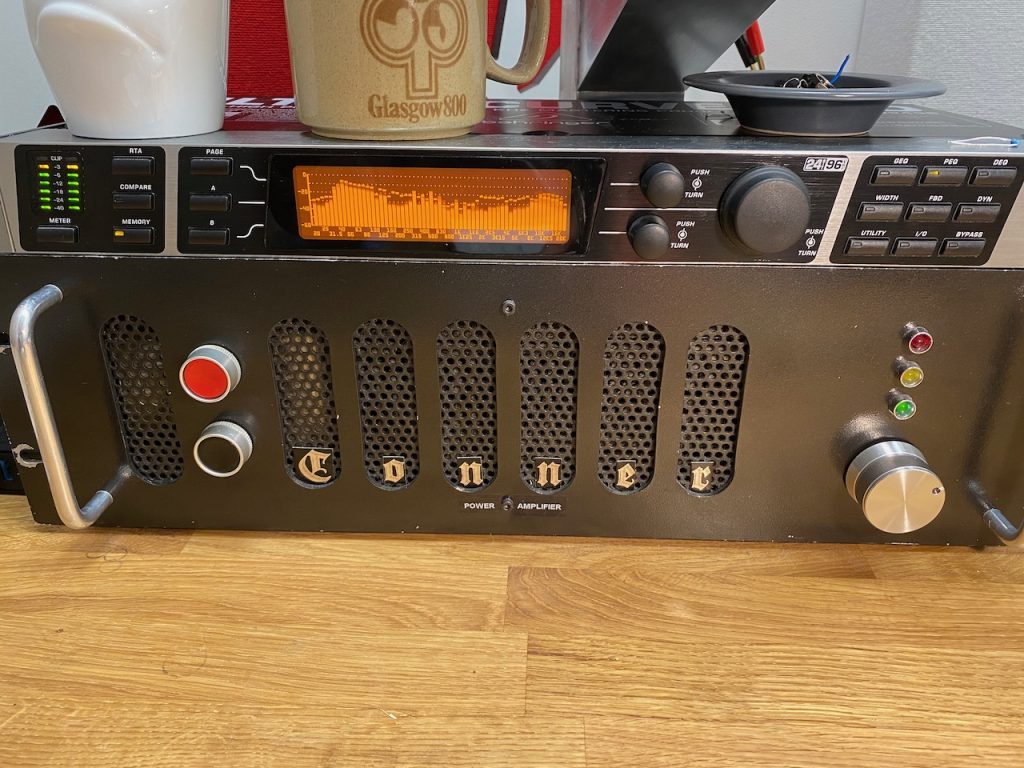
Now, you should never anthropomorphise amplifiers, they hate it! I swear that this one “wants” to blast electronic music from the 90s at high volume though. π
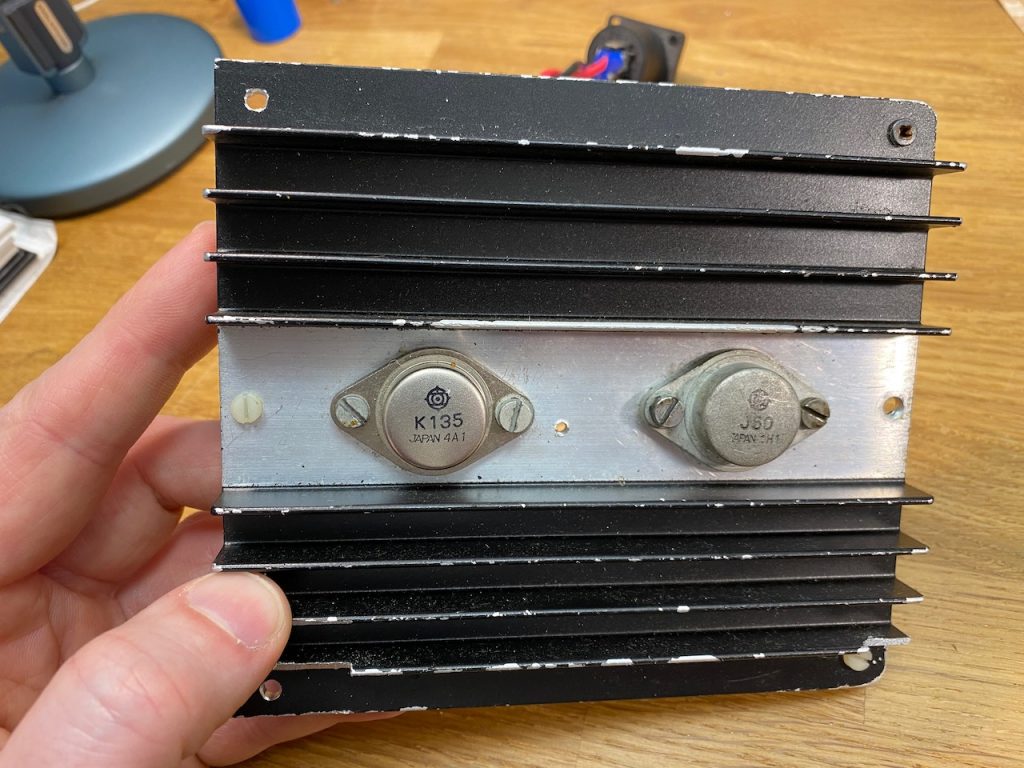
Having given the Ice Block its output stage back, I had to find another one for my experiments. A search of the junk pile yielded the remains of a Maplin 100W MOSFET amp kit. I’d have preferred to try BJTs, but the PG508 prototype was already set up to work with lateral MOSFETs.
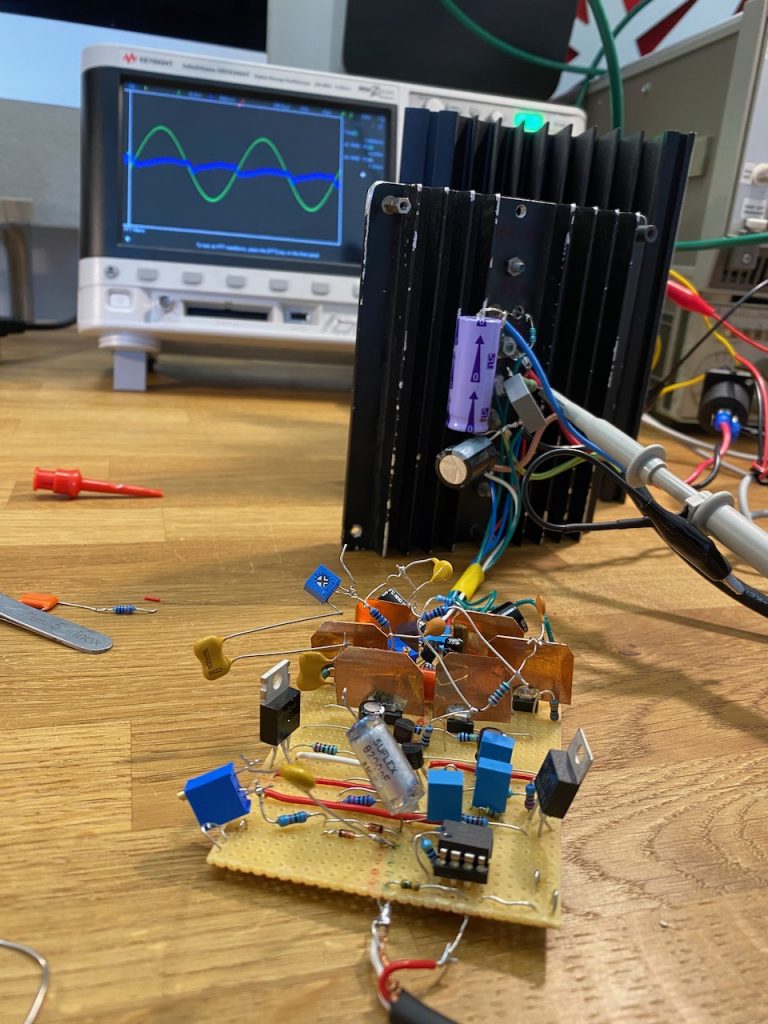
I tried the time-honoured method of soldering RC networks in random places, or maybe places that seemed to make a difference when touched with a damp finger. π This improved it somewhat, but it still wasn’t doing a great job of correcting the output stage’s copious (and vintage correct!) crossover distortion.
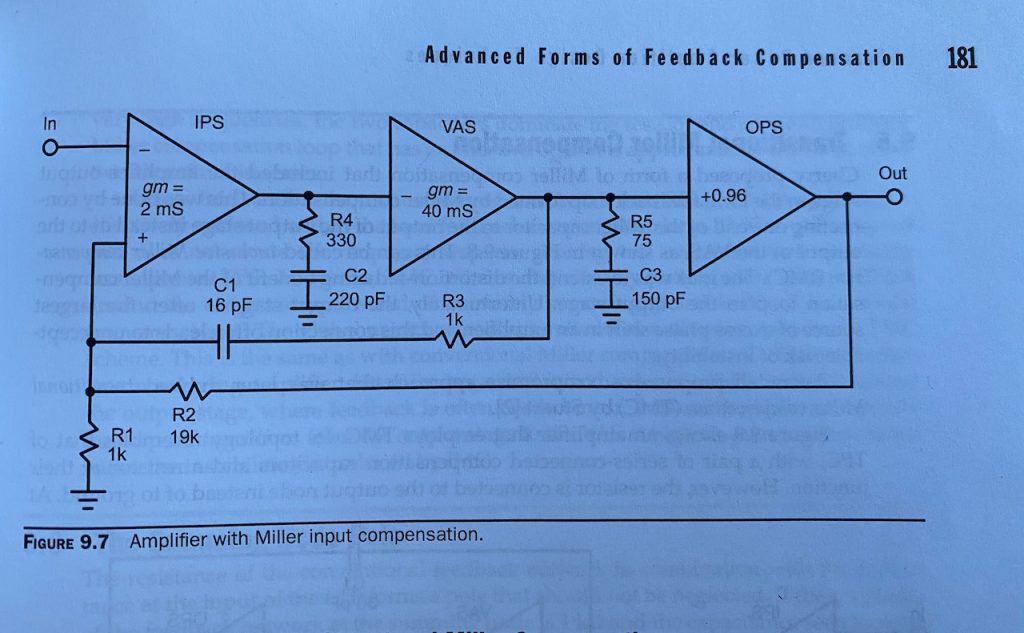
I eventually cracked Bob Cordell’s “Designing Audio Power Amplifiers” and spent an afternoon pondering Chapter 9, “Advanced Forms of Feedback Compensation”. It struck me that the PG508 topology is very similar to figure 9.7, except that the input stage doesn’t exist as such: the feedback node is the VAS input.
It also struck me that I’d already ended up with RC networks in the places shown in fig. 9.7 by trial and error, just with completely different values. R4 and C2 were in the original Tektronix PG508, and R5 C3, R3 C1 were my additions. So the obvious course of action was to change them to the values suggested by Cordell and see what happened.
Initial results weren’t great: it oscillated at 20MHz, but this was squelched by reducing R4 to 51 ohms. Having done this, performance was excellent: the 16pF C1 gave the extra loop gain I was looking for. I’d started out with 100pF here as that’s the value used in a Douglas Self Blameless amp. The Blameless input stage typically has 5-10x the gm of the PG508’s non-existent IPS, though. So funnily enough C1 needs to be 5-10x smaller to get comparable loop gain.
With these modifications the measured performance was 0.03% THD at full power at 10kHz, and 0.00something at 1kHz. The 10kHz figure seems high, but it’s now in the ballpark for a well functioning driver doing its best with a vintage MOSFET output stage. (Cordell’s AES paper quoted 0.02% at 10kHz with the Hawksford error correction turned off.)
Note that this THD figure is no better than I got with the old compensation and the Ice Block output stage. This just means that the Ice Block output stage must have about 3-5x less distortion than the single 2SK135/2SJ50 pair used here.
Slew rate was also improved, and stability with a capacitive load was just about acceptable: with 0.1uF slapped on the output it showed a few cycles of damped ringing but didn’t oscillate.
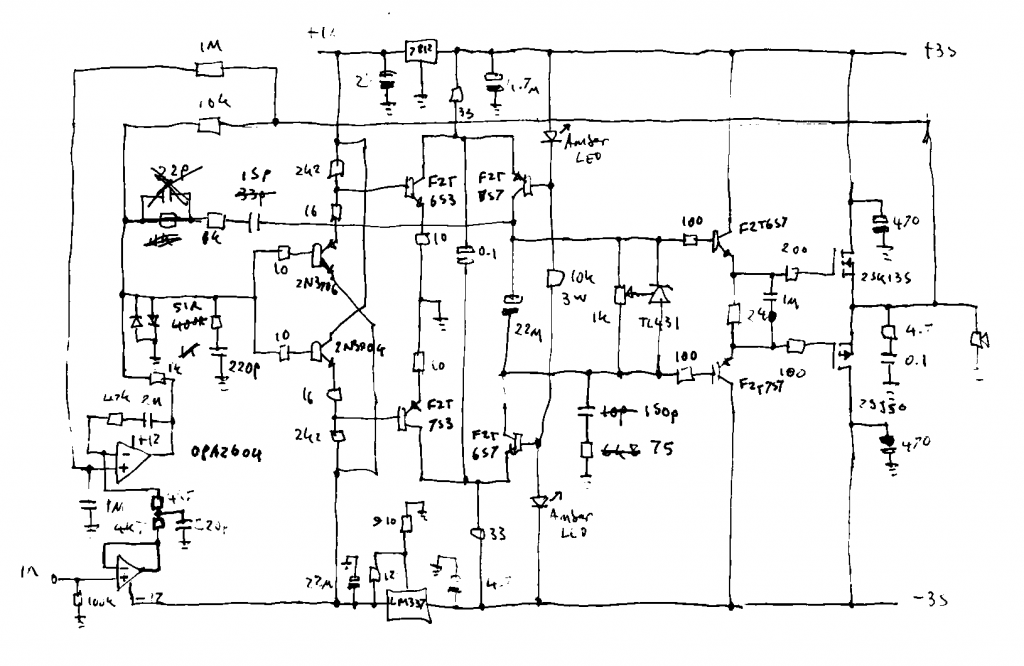
I also took the opportunity to test out the opamp front end inspired by the Quad 405 and Cordell Super Gain Clone. I used an OPA2604 as it was the best opamp I had around. This works very nicely: it reduces the DC offset to 2mV, undoes the phase inversion inherent in the PG508 circuit, and increases the overall gain from 10 to 50.
Note that the opamp must be a FET input type because of the high impedance of the DC feedback path. Also, as the circuit has 2 LF time constants (the 1M/1u and the 47k/2u) with feedback around them, it functions as a 2nd order active high pass filter. It rolls off at 12dB/octave and can resonate if the time constants are too close together.
I basically copied this part from the Quad 405, so it must have done the same thing. I guess it was desirable to have a good rumble filter here in the days of vinyl. Arguably it still is in the era of small vented speaker cabinets and dubstep. π